Karl Deisseroth: Using optogenetics to reveal the circuitry of depression in the brain
Karl Deisseroth: Using optogenetics to reveal the circuitry of depression in the brain
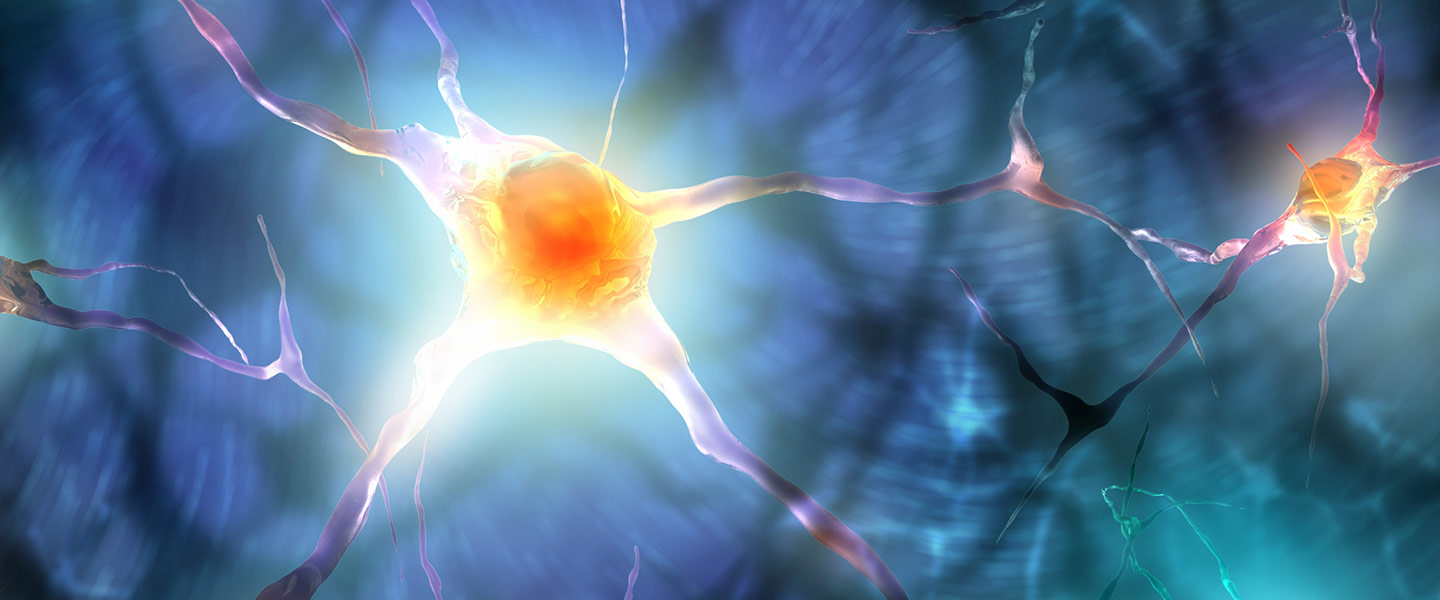
Stigma persists. Yet we are fortunate to live in an era in which major depression and other serious mental illnesses are considered just that — biologically-based malfunctions of the brain and nervous system, and not marks of personal failing or weakness.
What goes wrong at the level of nerve cells and circuits constitutes the current frontier of neuroscience. Scientists are pushing the boundaries of that frontier daily, making progress in thousands of projects at a pace that paradoxically aggravates and thrills.
For those afflicted and their loved ones, progress is understandably always too slow. For those performing experiments and connecting the myriad dots in the puzzle that is the human brain, the pace is nothing short of breathtaking, knowing as they do how quickly their understanding of fundamental processes is changing.
Few neuroscientists have done as much in recent years to illuminate how the brain works than Karl Deisseroth, M.D., Ph.D., whose career, by his own account, was jolted into fast-forward mode by a BBRF Young Investigator Grant. Less than a decade into his professional life, he is the inventor of a technique that has given rise to an entirely new field in neuroscience, which he has named optogenetics. In brief, it involves the use of light to rapidly open and close the membrane channels that make neurons fire and cease firing. With this tool, new vistas have been opened onto the mechanisms that give rise to depression and other brain and nervous system dysfunctions.
“I got a BBRF Grant very early on, and it had a big impact, in part because it came so early. The credibility that grant gave us had a disproportionately large effect. It really launched us.”
Deisseroth’s success in science has been meteoric. Several dozen scientific papers under his name have appeared in the leading scientific journals since 2005, and in addition to major prizes and accolades from the scientific community awarded to Deisseroth, his innovations have been written about widely in the mainstream press.
Like all of science, optogenetics builds on prior knowledge. For many years, microbial biologists had known that certain microorganisms like green algae can make light-activated proteins. Among these proteins are channelrhodopsins, tiny opening and closing gates whose activation enables the tiny organisms to move toward light. Deisseroth had the idea that these proteins (for example, one member of the family called ChR2) might be introduced into a nerve cell in mammals. If the protein could be localized to the cell’s membrane, it might then be possible to activate that neuron simply by shining a beam of light into the receptor.
The excitement over ChR2 is that it provides a way to manipulate a single type of neuron while affecting no others, an unprecedented specificity. Moreover, Deisseroth’s team knew that light, if it were of just the right wavelength, could open or close the ChR2 “gate” in precisely the time-scale in which neurons fire — over a span of thousandths of a second, the “millisecond scale.”
“The essence of optogenetics,” Deisseroth says, “is that it enables us to use light to make neurons active, and it does so in the language that the brain itself uses. Think about the heart: its ‘language’ is to deliver one pump every second or so. Well, if you can imagine a thousand different words or syllables being spoken in a second, you have an idea of the language of the brain. You need to operate at that speed to understand it and to communicate with it.”
“With optogenetics, you actually deliver the signal you want to the cell or cells that you want, and then see what the effect is on an animal’s behavior”
Deisseroth’s team used a noninfectious virus to deliver the ChR2 gene into specific nerve cells in living mice and rats. By implanting a painless fiberoptic probe in the animal’s head, they delivered a cool blue light to the brain and succeeded in “opening” the ChR2 “gate” in specified neurons, causing them to fire. “We saw on our recording devices that the action potentials — the millisecond events that happen in neurons — occurred when and only when we delivered these brief flashes. It was one-for-one: one brief light pulse, one ‘syllable’ of neural language.”
Recent advances in brain imaging have provided critical insights into brain function, but they are passive technologies. “With optogenetics, you actually deliver the signal you want to the cell or cells that you want, and then see what the effect is on an animal’s behavior,” says Deisseroth. “This enables us to begin marching through all the different circuit elements of the mammalian brain, triggering behavior in order to see what the jobs of all these elements are.”
It is a short leap from that kind of work — which his lab and many others are now engaged in, using Deisseroth’s ChR2 method — to research in which optogenetics is used to interrogate the malfunctions in nerve-cell communication believed to be at the root of psychiatric illnesses. It’s now possible to try to create symptoms of specific illnesses in living model animals “by driving the circuit, via optogenetics, in the wrong pattern” and observing the consequences, Deisseroth says. “We can also try to correct these patterns, perhaps by delivering the correct impulses to the correct cell types. In fact, we’ve already begun to explore this for patients with Parkinson’s disease.”
The neurotransmitter dopamine is well known to be dysregulated in Parkinson’s, but, asks Deisseroth, “what exactly is dopamine doing for the brain circuits” involved in the illness? “It’s a fundamental mystery that experimentation with electrodes and chemicals has not been able to solve.” Delivery of signals via electrodes — an experimental method called deep brain stimulation (DBS) — has shown some ability to moderate symptoms in Parkinson’s, although exactly why remains unknown. The same is true of the use of DBS in refractory major depression, a treatment method pioneered by Distinguished Investigator Helen Mayberg. When delivered to a spot in the cortex called area 25, DBS has alleviated symptoms in an encouraging percentage of study subjects. This has prompted Deisseroth to apply optogenetics and other methods to the study of depression and its still mysterious mechanisms.
The Deisseroth team made headlines in 2007 when they used a technique called voltage-sensitive dye imaging to reveal what’s going on in the brain of a depressed rat. Thomas Insel, M.D., head of the NIMH, called this a “stunning demonstration” of new technology whose ability to capture circuit dynamics in high resolution and in real-time — in the form of a movie — was likely to help build a “foundation for understanding depression as a brain disorder.”
Deisseroth used optical methods to get a rat’s brain cells to glow in response to electrical discharges that cause them to fire. This revealed that the glowing or “active” region in a part of the hippocampus called the dentate gyrus was smaller in depressed rats than healthy rats; and that the glowing area grew in size when the depressed animals were treated with Prozac. In these same animals the team also imaged the birth of new neurons, supporting other evidence that antidepressant treatment stimulates neurogenesis. Critically, though, when they suppressed neurogenesis in the dentate gyrus, rats did not become depressed. The birth of new neurons could account for the success of antidepression treatment; but its absence could not, in itself, cause depression. “We concluded that depression and depression treatment operate with different mechanisms at the cell level,” says Deisseroth; “but they do share circuitry — a common pathway.”
Using this and optogenetic techniques, Deisseroth and his colleagues will attempt “to understand at a much deeper level the ‘players’ in the circuitry of depression — which are important, what agents they work with to promote activity of the circuit, as well as inhibition of it. Do they operate abnormally only under certain conditions? If so, what are those conditions?”
He is talking about gaining a picture of depression at the level of its biological foundations, inside the tangled web of circuits that form the human brain. “We’re looking at interconnections between the hippocampus and other brain regions, and trying to understand what we might call a failure of communication, within and between regions.”
Featured Q&A With Dr. Deisseroth: