Team Develops an Innovative, Implantable Ultrasound Device to Stimulate Neurons in Deep-Brain Regions
Team Develops an Innovative, Implantable Ultrasound Device to Stimulate Neurons in Deep-Brain Regions
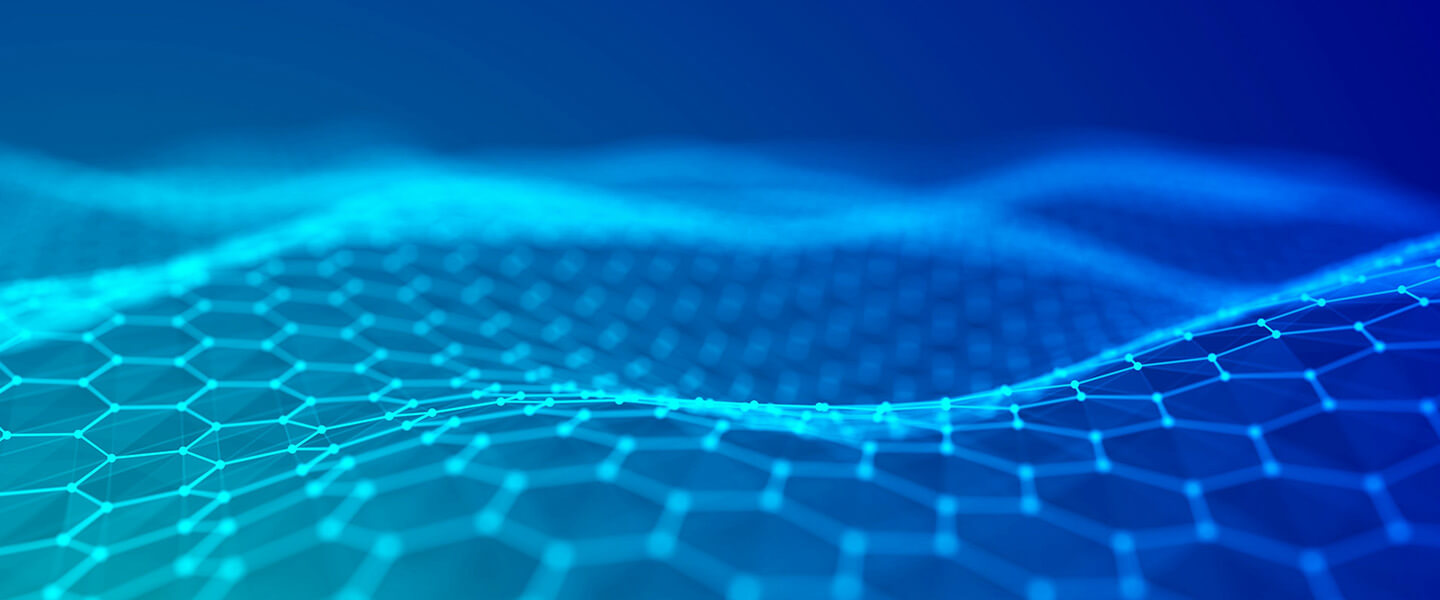
A research team led by 2018 BBRF Young Investigator Canan Dağdeviren, Ph.D., of the Massachusetts Institute of Technology, reports in Nature Communications that it has designed, developed, and successfully tested a tiny, implantable neurostimulation device that uses ultrasound to modify the activity of neurons deep in the brain.
Although still very much an experimental device, their neural stimulator, called ImPULS, in the team’s view has promise to become “a potent neuromodulatory tool” for therapeutic applications in people in illnesses ranging from major depression to Alzheimer’s. It may also prove useful in basic research on the brain.
ImPULS stands for “implantable piezoelectric ultrasound stimulator.” Ultrasound consists of sound waves that vibrate at greater than 20,000 cycles per second (20 kHz), a frequency that is very close to the upper limit of human detection. Many animals can hear in ultrasound wavelengths—from dogs and cats at the lower end to dolphins at the higher end. Ultrasonic waves with much higher frequencies are used for a wide range of medical applications, perhaps the most familiar being the visualization of the human fetus during pregnancy.
ImPULS is not the first device that uses ultrasound to stimulate the brain and alter the activity of neurons. Ultrasound has also been used, so far on a limited basis, to stimulate the brain non-invasively. In transcranial-focused ultrasound (tFUS) treatments in depression, Alzheimer’s and epilepsy, low-intensity ultrasonic waves are transmitted through the skull. Unlike the most common form of non-invasive neurostimulation, transcranial magnetic stimulation (TMS), which uses magnetism rather than sound waves to alter neuronal activity, tFUS has the advantage of being able to reach much deeper into the brain. It is thought ultrasound exerts its effects by affecting the tiny pores called ion channels that regulate the electrical activity of neurons. tFUS beams can be precisely focused (on the scale of millimeters), and penetrate several centimeters into regions far “beneath” the brain’s cortex, which lies immediately below the skull. Structures in the deeper subcortical regions include those such as the hippocampus and amygdala that play a central role in mood, memory, and learning.
Yet, as the MIT-led research team notes, “ultrasound, when transmitted from outside the human skull, faces significant scattering and reflection.” This can cause the stimulation of brain areas beyond the therapeutic target(s), and in some cases can potentially cause damage to the brain. These unintended “off-target” impacts are among the chief motivations for the MIT team’s work. Dr. Dagdeviren’s 2018 Young Investigator grant supported her work on developing a new, implantable interface that could precisely target areas of the brain known to be involved in Parkinson's disease. The current project is related to that effort, in that it also seeks to develop and test a device that can be surgically implanted in the brain to deliver ultrasound with a specificity and precision that exceeds what is possible in tFUS and other non-invasive ultrasound applications.
Implantable technology to deliver neurostimulation is also not a new idea. Helen S. Mayberg, M.D., a BBRF Scientific Council member, prizewinner, and 3-time grantee, pioneered DBS, or deep-brain stimulation, in the 1990s, to treat refractory depression. DBS involves the surgical implantation of electrodes and a device to supply power for stimulatory pulses. These have had dramatic and enduring results in a limited number of patients. Optogenetics, a technology pioneered by BBRF Scientific Council member and grantee Karl Deisseroth, M.D., Ph.D., uses beams of colored laser light delivered through very thin probes implanted in the brain to alter the activity of specific neurons, but has not been applied in humans in part due to its reliance on genetic manipulation of nerve cells, which is not yet feasible in people.
“A miniaturized, non-genetic platform for localized stimulation is therefore needed to fill the gap for next-generation neural interfaces to reach high standards of safety and longevity,” the MIT team says. Some early attempts at making ultrasound devices that fit this description have been proposed, but they may not be suitable for implantation deep in the brain “due to their rigid form factors, material composition, or high power requirements,” the researchers say.
ImPULS, the implantable piezoelectric ultrasound device they developed, is innovative in several respects. It has no active electrochemical elements. It is highly miniaturized, engineered at the micron-scale (1000 microns = 1 millimeter), is biocompatible, and uses very little power. Piezoelectricity is the electric charge that accumulates in certain solid materials, such as crystals, certain ceramics, and biological matter, in response to applied mechanical stress. To deliver the ultrasound, ImPULS uses a piezoelectric ceramic material micromachined to be only 30 microns thick, with an active element 100 microns in diameter. It is designed to be implanted in deep-brain regions, where its emission of ultrasound energy alters the behavior of adjacent neurons. In tests, the device resisted significant electrical and mechanical degradation over a period of days and did not raise the temperature of brain tissue during ultrasound generation beyond safe levels.
For the initial tests described in their paper, the ImPULS device was connected to an external printed circuit board via a special cable. The extremely thin probe whose implanted 100 micron-wide tip delivers the ultrasound energy was used in the laboratory to excite neurons in a preserved slice of mouse hippocampal tissue. Then, implanted deep in the brain of an anesthetized mouse, ImPULS was used in the living setting to prompt neurons to express a specific gene called c-Fos. Perhaps most intriguing, ImPULS was used in living mice to stimulate neurons that release dopamine in a part of the brain called the substantia nigra pars compacta. Careful application of ultrasound enabled the team to modulate dopamine release over a specific period of time. In Parkinsons’s disease, large numbers of dopamine neurons in this region at the back of the brain die or cease to function.
The team says the fabrication process enables them to scale ImPULS devices to target larger areas of the brain, if wanted. In future studies, they seek to gain finer control of neural stimulation and evaluate potentially distinct effects such as excitation vs. inhibition in a variety of cell types, neural circuits and brain regions. The team also hopes to produce versions of the device that can deliver ultrasound carrying greater energy. They will also study the durability of the device, hoping to demonstrate that it can survive a month-long implantation. Still other research will try to adapt the technology for specific basic research projects seeking to better understand brain function.
“We believe this implanted ultrasound stimulation device can be developed into a versatile tool for both basic systems neuroscience research and potential therapeutic applications,” the researchers said.
Steve Ramirez, Ph.D., a 2016 BBRF Young Investigator, was a member of the team.