Insights Into Sensory Symptoms in Autism and Other Disorders from Stem Cell-Based Model of Key Human Sensory Pathway
Insights Into Sensory Symptoms in Autism and Other Disorders from Stem Cell-Based Model of Key Human Sensory Pathway
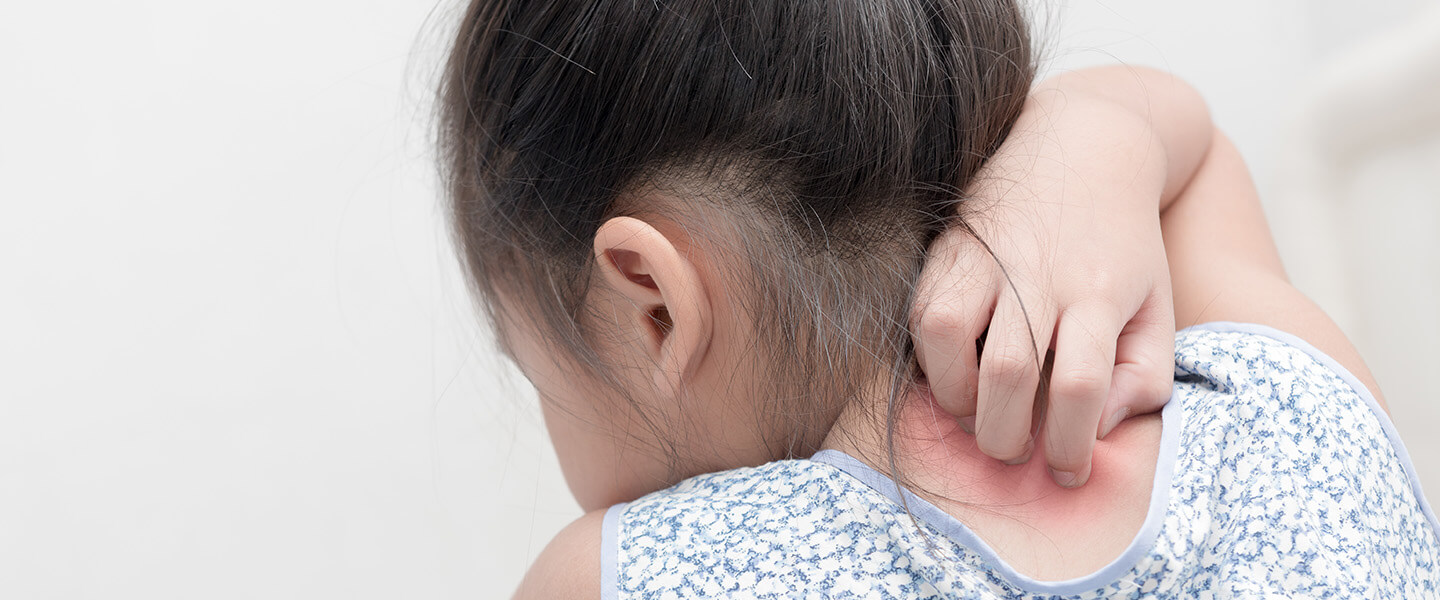
Sensory processing difficulties are one of the most prominent symptoms of autism spectrum disorder (ASD). Those affected may experience greatly heightened, or, in some cases, reduced sensitivity to a variety of sensory inputs, ranging from bright light and loud sounds to the rough texture of articles of clothing.
Problems with interpreting the signals coming into the brain from sensory organs is also a major issue in schizophrenia and bipolar disorder, in individuals who experience psychosis. Those diagnosed with ADHD may experience sensory issues, including hypersensitivity to certain stimuli. Depressed individuals, as well, may experience sensory problems.
While sensory symptoms related to psychiatric disorders are well documented, treatments for the illnesses mentioned do not specifically address them. It is also true that while a great deal is known about our sensory organs and about vital faculties like hearing, vision, smell, and touch, relatively little is known about how genetic or environmental disruptions of sensory pathways can give rise to sensory symptoms. This is especially true for pathologies in ASDs, for instance, relating to the senses that emerge at the dawn of life, when the brain is developing in the fetus and in the young child.
The discovery of relevant new treatments for sensory dysfunction “has been hindered by the lack of reliable human experimental models” of pertinent sensory pathways, say authors of a new paper appearing in the journal Nature. Led by two-time BBRF grantee Sergiu P. Pasca, M.D., of Stanford University, the investigators reported their success in building just such a model—a functional model of the human neural sensory pathway.
This pathway is crucial for conveying a wide range of sensory information (including pain) as it moves from the body’s periphery, into the spinal cord, then into a region of the brain called the thalamus, and on to the outer layer of the brain—the cerebral cortex. Probing of all the components of this circuity “has not yet been achieved in animal models,” the team says, “which limits our understanding of the consequences of various sensory manipulations at the circuit level.”
The team made use of stem cell technology to build their model of the human sensory pathway. Dr. Pasca is among the pioneers who in the last 15 years have developed ways of taking skin and blood cells harmlessly sampled from people (including psychiatric patients) and returning them in the laboratory to a stem cell-like state. The remarkable technology involves not just returning a skin or blood cell back to a stem-like state, but then guiding such cells to become specialized cell types—neurons or glial cells in the brain, for example.
Using this technology, called human induced pluripotent stem cell technology (hiPS), Dr. Pasca’s team has figured out how to grow large numbers of reprogrammed cells to form structures called brain organoids, which differentiate, self-assemble, and, remarkably, “wire up” so that the cells which start out in a primitive state such as is typically seen in the developing brain, form connections. Organoids made of human cells have been implanted in the brains of rats and assume functional roles specific to the regions in which they are implanted. Organoids can also be combined, as shown for the first time by Dr. Pasca’s team, to form multi-unit complex structures that are now known as “assembloids.”
The functional model of the human neural sensory pathway just reported by the team is an assembloid composed of four organoids. Each reconstitutes a part of the full pathway, and was created separately, before all four were integrated to form a functioning unit. The four parts (in order of “ascent” in the actual human pathway) include organoids containing key cell types found in the somatosensory system (sensory organs); the dorsal spinal cord; the thalamus; and excitatory neurons of the cerebral cortex.
Reconstructing this pathway and its circuits ex vivo—outside of a living body, in the lab—is a powerful tool, the team reports, “holding significant promise for understanding the mechanisms involved in the development of the human sensory system and for unraveling, therefore, the pathophysiology of sensory-related disorders.”
This four-part assembloid enabled the team to visualize transmission of calcium signals through the entire range of the network following the application of a chemical sensory stimulus. They were able to capture properties of the full sensory pathway as it functioned as a unit in ways that could not be seen in the individual organoid components prior to their integration. For example, following delivery of the chemical stimulus, the team could observe how the four assembloid components operated in a coordinated way.
The team was able to observe functional consequences when they performed specific manipulations in cells within the assembloid. When they experimentally deleted a type of sodium ion channel—a tiny pore in neurons through which charged atoms of sodium pass in and out of the cells—they noted a disruption of synchrony throughout the network. A different manipulation, involving expressing a known gene variant, led to observations of hyper-synchrony. These two manipulations showed how manipulation of cells in the assembloid could affect the whole so as to generate pathologies linked, respectively, to insensitivity and extreme sensitivity to pain.
“Going forward this multicellular platform can be applied to a wide range of disorders including sensory dysfunction in neurodevelopmental disorders or hyperalgesia (extreme pain sensitivity), and could be useful for drug screening” the researchers said.
The team hopes to improve the sensory assembloid model by developing ways to have component neurons express a greater variety of receptors, which would expand its repertoire of responses to sensory stimulation, such as itch, pressure, or altered temperature. Another aim would be to build other neural pathways involved in sensory perception, to be mobilized in parallel with the current model or perhaps through integration with it, so as to be able to model even greater complexity in the system that senses and transmits sensory information to the brain for processing. A particular interest is in how these vital circuits evolve from very rudimentary beginnings in the first stages of life—the time when it is thought that dysfunctions begin which may culminate in a range of sensory symptoms after birth, for instance, in autism.
In addition to Dr. Pasca, who is a 2017 BBRF Independent Investigator and 2012 Young Investigator, the team included Kevin W. Kelley, M.D., Ph.D., 2024 BBRF Young Investigator; and Neal D. Amin, M.D., Ph.D., 2021 BBRF Young Investigator. The paper’s co-first authors were Drs. Ji-il Kim and Kent Imaizumi.